Science has come a long way in helping us understand the way the brain changes in addiction. In this section, we will provide updates of research on how the brain is impacted during addiction and recovery.
Click to Learn more about: Neurotransmitters, Agonists, and Antagonists3 Key Points to Understand the Brain and Addiction:
1. Some characteristics of addiction are similar to other chronic diseases.
Just as cardiovascular disease damages the heart and alters its functioning, addiction changes the brain and impairs the way it works. Below is a comparison image of the brain (top) and the heart (bottom).
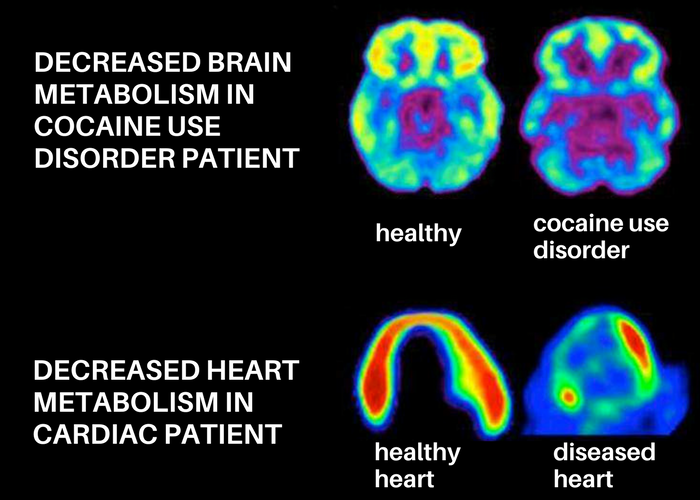
These scans demonstrate how scientists can use imaging technology to measure the functioning of different parts of the body. Greater activity is shown in reds and yellows, and reduced activity is shown in greens, blues, and purples. The healthy brain and healthy heart both show greater activity than a brain or heart that has been impacted by disease. In drug addiction, the prefrontal cortex in particular tends to be impacted and show changes in functioning. This is the part of the brain associated with things like judgment and decision-making, which causes individuals with substance use disorders to often have difficulties with impulse control related their substance use.
Ultimately, much like other chronic diseases, addiction is preventable and treatable, but it does change one’s biology and can last a lifetime if it goes untreated.
2. Substance use tricks the brain’s reward system.
The brain experiences pleasure from many different things we like to do in life; eating dessert, having a sexual encounter, playing a video game, etc. This pleasure is signaled through the release of the neurotransmitter dopamine (a chemical messenger present in the brain’s connected pathways) into the nucelus accumbens, which is the brain’s pleasure center. Generally, this is a positive thing, as it ensures people will receive pleasure from doing things necessary for survival.
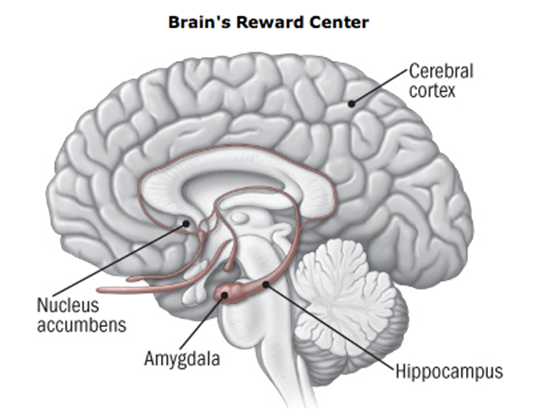
However, dopamine is also released into the nucleus accumbens when using substances like nicotine, alcohol, and heroin. In addition, addictive drugs can release significantly more dopamine that non-drug-related rewards do, and they do it more quickly and with more regularity. This means that addictive drugs can create a shortcut to the brain’s reward system by flooding the nucleus accumbens with dopamine.
Over time, the brain becomes more resistant to dopamine (an effect known as tolerance) to try and account for the excess that is produced when using drugs, and this lessened effect means that the drugs will become less rewarding as craving increases. Individuals who develop a substance use disorder will find that over time the substance will no longer give them the same amount of pleasure as before, and they subsequently have to take larger amounts of the substance more frequently to feel an effect.
There is a difference between “liking” and “wanting” a substance. Over time, “liking” the drug decreases, but “wanting” or craving the drug increases. Other non-drug rewards that someone might have enjoyed before will also be impacted by the tolerance effect, and will produce less pleasure over time. Individuals with substance use disorders continue to seek and use the substance, despite the associated negative consequences and harms caused both for themselves and their loved ones, because the way the brain’s reward system has been altered means that the substance allows them to simply feel more normal.
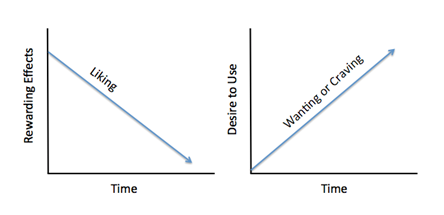
3. The brain can recover – but it takes time!
The neuroscience of addiction recovery is an exciting and emerging area of research. There is evidence that the brain does recover over time; this image below shows the brain of a healthy control on the left, and a patient in recovery from methamphetamine use disorder on the right. After one month of abstinence, the patient’s brain is clearly showing lessened activity than the healthy control. However, after 14 months of abstinence, their brain is returning to a more healthy baseline, and the dopamine transporter levels (DAT) in the reward center of the brain are able to return to a nearly normal level of functioning.
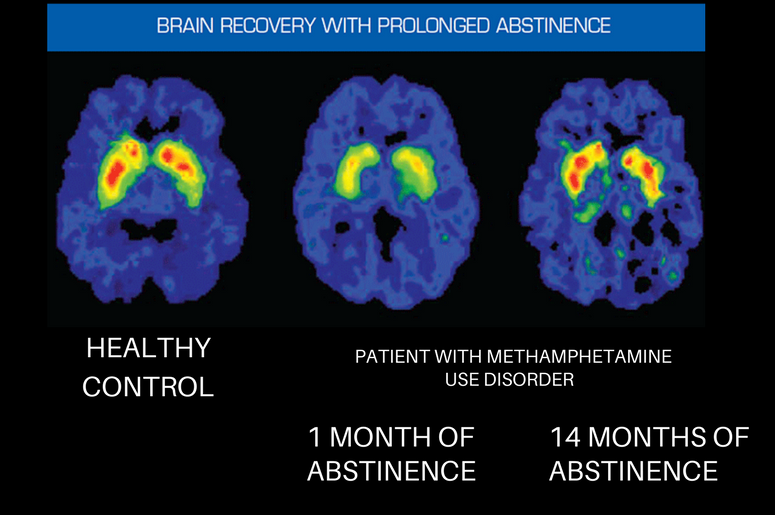
Currently, there is still relatively limited research on the brain’s recovery from alcohol and cannabis use. However, research is beginning to show that some amount of recovery does take place. For example, studies have shown that adolescents who became abstinent from alcohol use showed significant recovery in regard to behavioral disinhibition and negative emotionality. Lisdahl and colleagues propose that this could indicate a return to near normal functioning in the prefrontal cortex after a period of abstinence, similar to the image example shown above. Furthermore, other studies have demonstrated that a higher number of days abstinent from alcohol was associated with improved executive functioning and larger brain matter volume.
While these results are promising, this field of research is still fairly young with new studies coming out regularly, and there have also been conflicting results that show minimal to no recovery from cognitive deficits after alcohol and cannabis use. This is particularly noteworthy for studies evaluating the brain’s recovery from cannabis use. On the other hand, some studies have shown that abstinent cannabis users demonstrated increased cognitive functioning and executive functioning than non-abstinent users. Whether this is associated with a compensatory response or neural recovery is still being investigated.
What is clear is that alcohol and cannabis do have some neurotoxic effects and that, at least to some degree, this damage is able to be reversed in a similar way that the brain is able to recover from using other substances. Research on how we can specifically improve brain recovery from substance use is still ongoing as well, but emerging literature suggests that physical exercise could be beneficial in this way by improving neuroplasticity, which is the resiliency of the brain to adapt to harm and adjust neural pathways to allow for normal functioning after being damaged. Previous studies have demonstrated that physical activity is able to improve cerebral blood flow, white matter integrity, and executive control. Further studies examining related interventions in adolescent alcohol or cannabis users will need to be investigated further.
The Top Tools Being Utilized for Research on the Brain in Recovery
Functional brain imaging techniques:
Methods that can demonstrate dynamic physiological information about brain function and activity. Functional imaging techniques allow researchers to measure the contributions and impact of specific brain structures to specific psychological processes (e.g., attention, working memory, impulse control, etc.). This imaging is usually performed while participants complete specific tasks, which allows researchers to identify brain regions that are activated, or recruited, to perform said task. Atypical brain function in clinical populations may include reduced neural activation or a different pattern of neural activation when performing certain tasks as compared to a health control population.
- Functional Magnetic Resonance Imaging (fMRI)
-
Also known as a functional MRI (fMRI), this imaging technique measures brain activity by detecting changes in blood flow and oxygenation.
- Numerous studies utilizing functional magnetic resonance imaging (fMRI) have shown that drug cues elicit increased regional blood flow in reward-related brain areas among addicted participants that is not found among controls
See the fMRI in action:
- Electroencephalography (EEG)
-
An electroencephalogram (EEG) is a test that detects electrical activity and patterns in the brain using small, flat, non-invasive metal discs (electrodes) attached to the scalp. Brain cells communicate continuously via electrical impulses, even while asleep, and this activity is shown via fluctuating lines on an EEG recording.
See the EEG in action:
- Functional Near Infrared Spectroscopy (fNIRS)
-
Functional Near Infrared Spectroscopy (fNIRS) is an imaging technique that monitors changes in oxygen concentrations during neural activity by measuring the difference in absorption levels of near-infrared light (NIR) between the spectrum of 700-900 millimeters.
See the fNIRS in action:
- Positron Emission Tomography (PET)
-
Positron Emission Tomography (PET) is a nuclear medicine imaging technique that uses a radioactive drug tracer to detect how tissues and organs are functioning, measuring low concentrations of molecules to detect cell-to-cell communication, and track the distribution of a substance within, and movement into and out of, the brain.
See the PET in action:
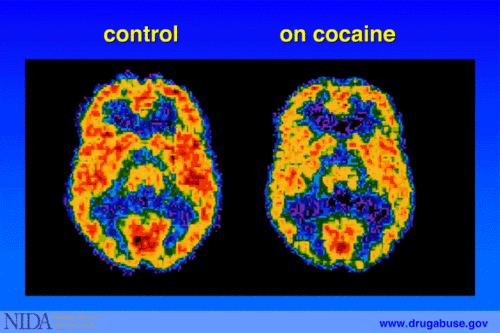
Structural brain measurement techniques:
Structural imaging techniques allow one to examine the brain’s physical, anatomical structure. Structural imaging is essentially cross-sectional, in that it provides static information and is analogous to taking a photograph of the brain. These images permit evaluation of overall anatomical abnormalities, including tissue atrophy (i.e., loss of neural tissue) and reduced white matter integrity (i.e., weakened connections between neural structures).
- Magnetic Resonance Imaging (MRI)
-
Imaging technique that uses a magnetic field and radio waves to generate detailed images of water molecules in a cross section or area of the brain. Different types of tissue hold different amounts of water, and this allows the generation of maps or pictures of the brain that contrast and detect structural abnormalities such as size, density, and volume of brain tissue (such as white matter or gray matter).
See the MRI in action:
- Diffusion Tensor Imaging (DTI)
-
This is an MRI-based neuroimaging technique that detects microstructural changes or diseases of nervous system tissue (neuropathology), characterizing the location and orientation of white matter through the generation of brain maps. These maps use contrasting colors to create an image that highlights the diffusion of water molecules through the brain.
See the DTI in action:
Limitations of brain measurement tools for addiction research:
- Utilizing brain imaging technologies in research studies can be very expensive.
- Some neuroimaging techniques require IV injection of a radioactive tracer (e.g., PET scan) or a contrasting agent (MRI).
- Some techniques are not suitable for everyone. For example, individuals with certain pacemakers or metal implants cannot undergo magnetic resonance imaging due to concerns with safety or accuracy of the imaging.
- Research methods must be tailored to imaging requirements. fMRI, for example, is sensitive to physical movement and requires that the individual being scanned remains as still as possible. Therefore, tasks performed during an fMRI scan must not require excessive movement for the scan to work properly.
- Different imaging techniques have different advantages or disadvantages. Some methods provide better temporal resolution (the accuracy of capturing an image with respect to time), whereas others provide superior spatial resolution (the visual clarity of the image). Although no single technique has perfect spatial and temporal resolution, multimodal imaging techniques (the simultaneous use of 2 or more techniques) are being more commonly implemented and can provide a more complete picture of brain structure/function than an individual technique.